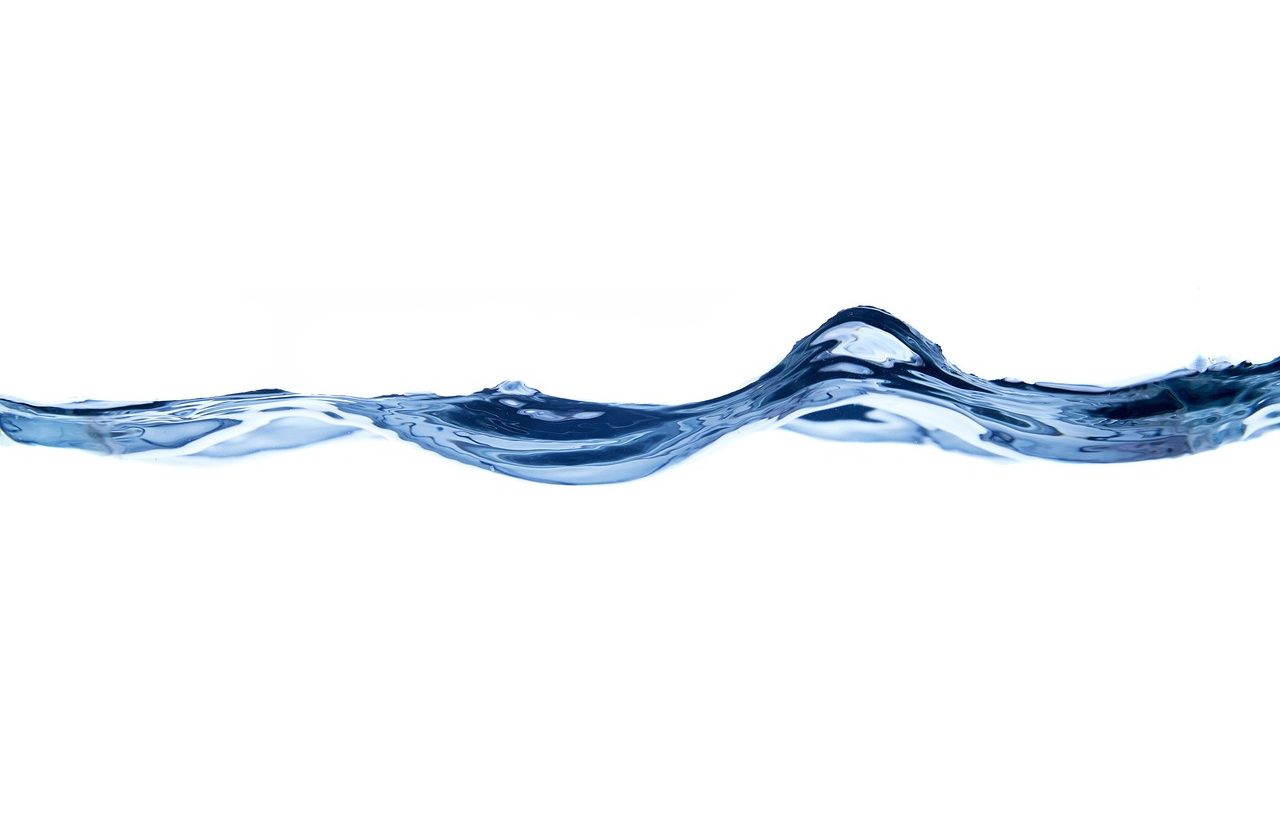
The tunnel effect occurs when the wave function extends beyond the barrier.
The tunnel effect is a quantum phenomenon in which a particle can cross an energy barrier that, according to the laws of classical physics, should not be able to overcome. This effect occurs due to the probabilistic nature of subatomic particles in quantum mechanics. A known example is that of electrons in tunnel diodes or nuclear fusion in the stars.
Theoretical Fundamentals
The tunnel effect is a quantum phenomenon that is observed when a particle crosses a potential barrier, something impossible from the perspective of classical physics. This behavior is explained from quantum mechanics through the concepts defined below.
Wave function
It is used to describe the status of a particle. It contains all probabilistic information about the position and moment of the particle. Although from a classic point of view a particle could not overcome a barrier if it does not have enough energy, its wave function can, although with few probabilities.
Potential barrier
A region where potential energy is greater than the kinetic energy of the particle. Although its height exceeds the energy of the particle, the latter is likely to cross it.
Quantum tunnel
Phenomenon thanks to which the wave function is not completely canceled when it reaches the barrier . This allows, on the other side, there is a finite probability to find the particle.
Heisenberg uncertainty principle
This principle establishes that it is not possible to know simultaneously the position and time of a particle. This indeterminacy contributes to the possibility of the tunnel effect, since the uncertainty in the position and energy of the particle allows, at the quantum level, escape from the classical restriction of the potential barrier.
Schrödinger equation
It is fundamental in quantum mechanics, since it describes how the wave function of a particle changes in time. In the context of the tunnel effect, this equation allows to calculate the probability that a particle crossing a potential barrier. Its general form is: Total energy of the particle = kinetic energy + potential energy .
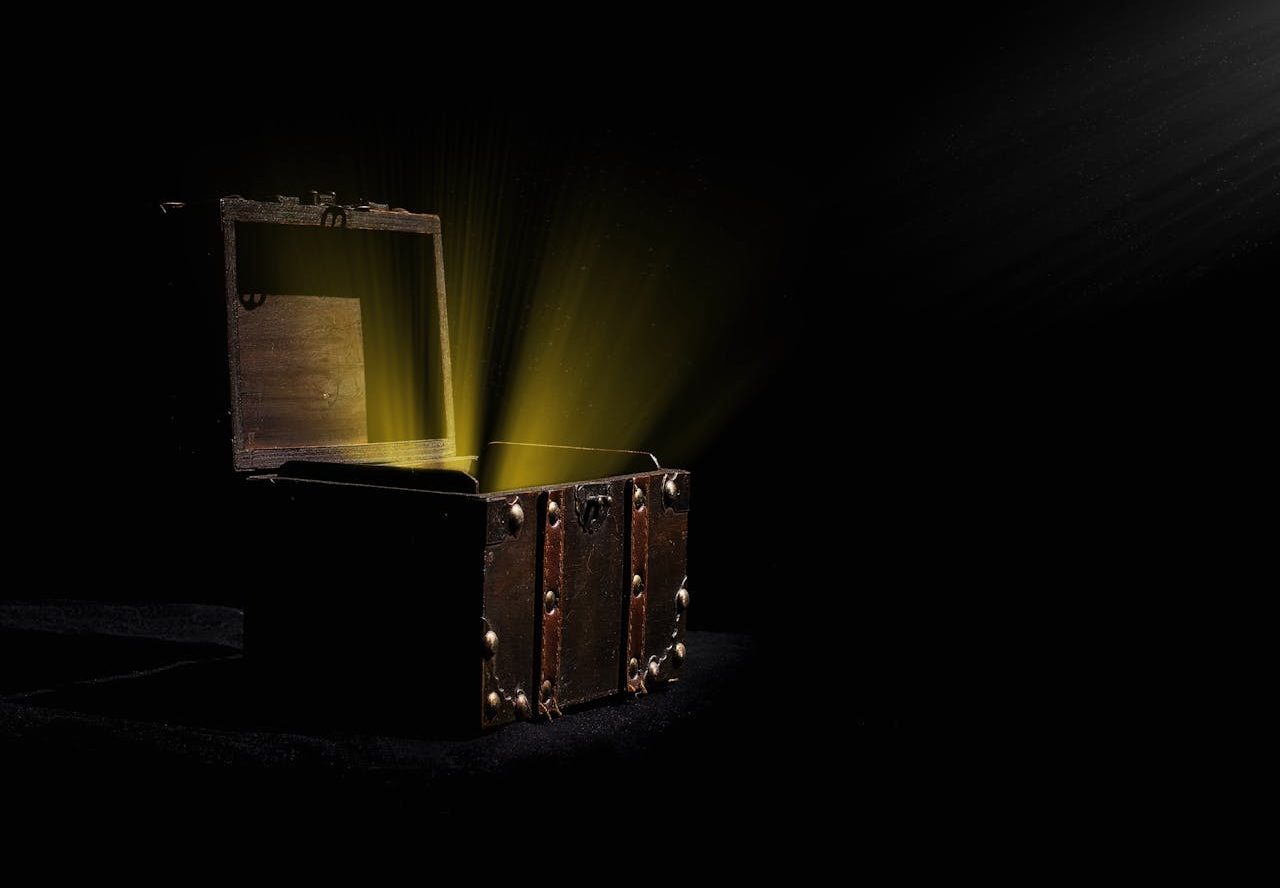
The particles are confined in a limited space with discreet energy levels.
Fundamental quantum phenomena
In quantum mechanics, several experiments and concepts help us understand the peculiar behavior of particles at a subatomic scale, where the rules of classical physics are not related in the same way.
Particle in a box
This is an idealized model that describes how a particle, such as an electron , behaves when confined in a limited space. In this situation, the particle can only exist at certain discrete quantum energy levels . Unlike classical systems, the energy of the particle cannot take any value, but is quantized into specific values.
Quantum superposition
Quantum mechanics allows particles to exist in multiple states at once, a phenomenon known as superposition. A famous example of this is the double-slit experiment , in which a particle can simultaneously pass through two slits and generate a wave-like interference pattern, highlighting its superposition nature.
Wave-particle duality
A fundamental principle of quantum mechanics that states that subatomic particles, such as electrons or photons, can behave both as localized particles in space and as propagating waves. This duality is key to understanding the behavior of particles in quantum systems.
Advanced aspects
As we delve deeper into quantum mechanics, tunneling is related to more complex phenomena and advanced theories that expand our understanding of the quantum universe.
Quantum entanglement
This phenomenon occurs when two or more particles are in a shared quantum state, so that the state of one instantly affects that of the other, regardless of the distance that separates them. While tunneling itself does not imply entanglement, both phenomena reveal the nonlocality of quantum behavior, where distant particles can influence each other beyond what is allowed by classical laws.
Quantum teleportation
Related to quantum entanglement, quantum teleportation is a process in which the quantum state of one particle is transferred to another at a distance, without the physical particle itself moving. While this phenomenon is not the same as quantum tunneling, both show how particles can overcome classical limitations of space and energy in surprising ways.
Quantum field theory
This theoretical framework combines quantum mechanics with special relativity, describing particles as excitations of quantum fields. In this context, tunneling can be understood not only as a phenomenon of individual particles, but also as a process of field fluctuations that allow the appearance of virtual particles that contribute to the dynamics of quantum tunneling.
string theory
In its quest to unify gravity with quantum mechanics, string theory proposes that the fundamental particles are not points, but small vibrating strings. Although tunneling is usually explained in terms of particles, string theory suggests that tunneling processes could involve the vibrations of these strings, leading to an even deeper understanding of the underlying quantum mechanisms.
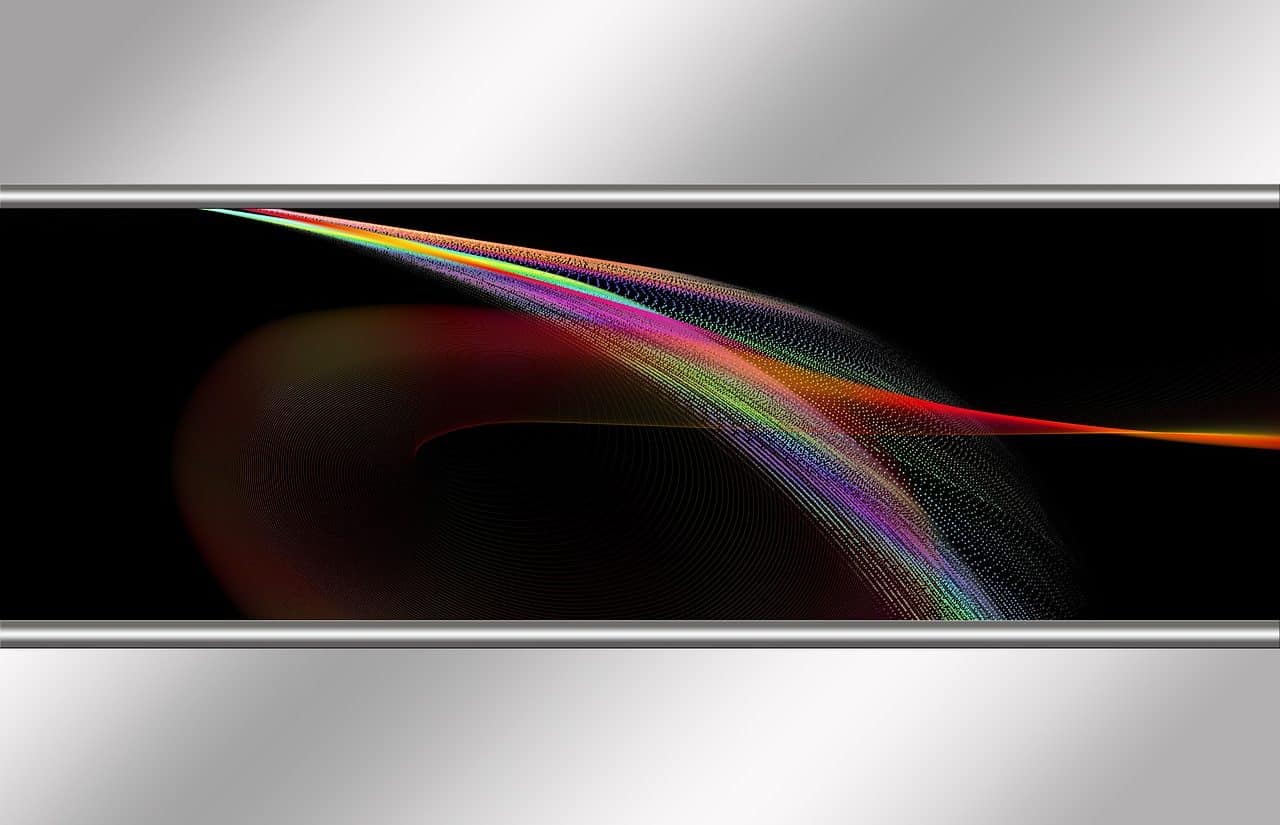
Quantum superposition allows subatomic particles to exist in multiple states simultaneously.
Practical applications
The tunneling effect is a theoretical phenomenon with crucial applications in modern technologies that are transforming fields such as electronics and computing.
Quantum electronics
Tunneling is the basis of several advanced electronic devices that are part of everyday life. For example, tunnel diodes and tunneling transistors take advantage of this phenomenon to improve the efficiency and speed of electrical current conduction through extremely thin potential barriers. These devices are essential in the miniaturization of electronic components, allowing the development of more compact and faster circuits, such as those found in microchips.
Quantum computing
In quantum computing, tunneling plays a key role in the operation and manipulation of qubits , the basic units of quantum information. Unlike classical bits, which can only be in a state of "0" or "1", qubits can exist in superposition of both states simultaneously, allowing for much more complex and efficient calculations.
Related quantum effects
Tunneling is linked to several quantum phenomena that illustrate the non-intuitive behavior of subatomic particles and the interactions between fields and particles.
Zeeman effect
It occurs when the energy levels of the electrons in an atom split in the presence of a magnetic field. While not directly related to tunneling, both phenomena involve changes in quantum energy levels based on external conditions.
Photoelectric effect
It occurs when light hits a material and releases electrons. It has similarities to the tunneling effect in terms of the release of electrons that would normally be confined. In both cases, these are particles overcoming a potential barrier.
Quantum Hall Effect
The electrical resistance of a material subjected to a magnetic field is quantized. Although it does not involve quantum tunneling directly, both effects share the discrete nature of energy levels and the influence of external conditions on quantum behavior.
Josephson effect
It is a direct example of quantum tunneling in which pairs of electrons can pass through an insulating barrier between two superconductors. It is essential in devices such as SQUIDs (superconducting quantum interference devices).
Meissner effect
The expulsion of magnetic fields from a superconductor when it cools below its critical temperature. Although the Meissner effect does not involve quantum tunneling, both reflect quantum behavior in the conduction of electricity and the control of magnetic fields.
Casimir Effect
It occurs when two very close metal plates experience an attractive force due to fluctuations in the quantum vacuum. Like quantum tunneling, the Casimir effect results from quantum phenomena that only manifest themselves at extremely small scales and that defy classical intuition.